Daylight Harvesting Lighting Controls
Using Artificial Light Only When Needed
Daylight harvesting can be as simple as manually switching lights off whenever there's enough daylight to meet an area's illumination requirements. This can save a substantial amount of energy, and is "free" in the sense that it requires no additional equipment.
Unfortunately, occupants of non-residential buildings aren't very disciplined about turning lights off even when leaving a room (which is why occupancy sensing light switches save so much energy). And they're even less likely to manually control the lights, in response to daylight levels, while remaining and working in the room.
In fact, occupant use of manual lighting controls (both switching and dimming) in office settings has been the subject of extensive research, which shows that manual controls are rarely operated other than at the beginning or end of the workday.
For example, after reviewing the extant literature and conducting their own experiments, a group of researchers concluded, "...These findings from independent studies conducted at different times on different continents with different levels of technology imply a common, stable pattern of behaviour. This is to use the switching and dimming lighting controls to set the light level to what is expected for the day's work at the beginning of the day, and then to change it only when essential" (Boyce at al. 2006, paragraph 3.1)
Fortunately, the technology to control lights automatically as a function of daylight level is available off-the-shelf at low cost.
Automatic Controls
Automatic daylight-havesting lighting controls can be grouped according to three major design characteristics:
- Auto-dimming versus auto-switching
- Closed-loop versus open-loop
- Integrated versus stand-alone systems
Auto-Switching versus Auto-Dimming
The earliest automatic daylight-harvesting lighting controls, developed decades ago, were exclusively of the auto-switching type. Such controls provide single-level or multi-level adjustment of the lighting level by switching one or more lamps on or off in response to the output of one or more photosensors. Modern switching-only controls are inexpensive and robust, but the step changes in light level they produce can be obtrusive to building occupants. And because the light level can only be changed in discrete steps, there is almost always either too much or too little light for the prevailing conditions.
Also available, today, are auto-dimming controls that smoothly and continuously regulate lamp brightness to maintain a nearly constant illumination level. Daylight sensed by one or more photosensors will cause such a control to automatically dim the lamp, saving energy. Auto-dimming controls offer additional benefits beyond daylight harvesting:
- Lumen maintenance. Because fluorescent lamps produce less light as they age, lighting systems are typically designed to produce excess light when the lamps are new, ensuring adequate brightness over the entire lamp lifetime. Unfortunately, the excess brightness initially wastes energy. By automatically regulating lamp brightness, some auto-dimming controls can eliminate the energy wasted when the lamps are young.
- Manual dimming capability. Because an auto-dimming lighting control is essentially a dimming ballast with a photosensor and special control circuitry, it inherently offers the potential for the same manually adjustable dimming capability as ordinary dimming ballasts. This is usually implemented via an "illumination thermostat" that occupants can use to set the preferred illumination level. While dimming capability can increase energy savings, the main benefit is increased occupant satisfaction. However, the user interface for the dimming function (either a wall-mounted switch or a handheld remote control) increases system cost.
On the other hand, auto-dimming controls have two significant disadvantages:
- Because they require (or, in come cases, incude) a dimmable ballast—and because most buildings are not already equipped with dimmable ballasts—they are significantly more expensive than auto-switching controls.
- System efficiency drops as lamps are dimmed. So, for example, at 10% brightness, the lighting system might still be consuming 30% of the power consumed at maximum brightness, offsetting some of the savings from the harvested daylight. The loss in savings can be substantial in areas which receive plenty of daylight.
In order to recover the savings lost due to dimming inefficiencies, some dimming controls are capable of automatically switching the lamps fully off when the daylight level increases beyond a certain point. These dimming-and-switching controls cost more than switching-only and dimming-only controls, but provide the greatest savings over a broad range of daylight levels.
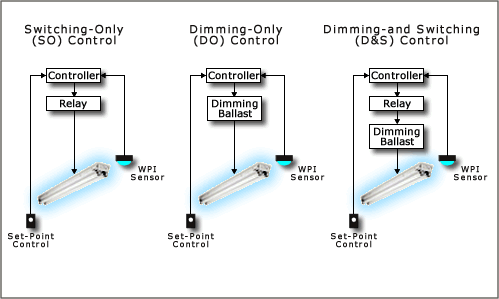
Chart 1: Switching-Only, Dimming-Only, and Dimming-and-Switching Lighting Controls
Closed-Loop versus Open-Loop Control
Automatic daylight harvesting lighting controls can be grouped into two categories, depending on exactly what their photosensors sense:
- In closed-loop control, the photosensor is located and oriented to sense a portion of the light produced by the controlled lamp, as well as any daylight present in the daylit zone. The system adjusts the dimming level (or switches the lamps) to maintain the desired total illumination, as directly sensed by the photosensor.
- In open-loop control, the photosensor is located and oriented to sense admitted daylight, but virtually none of the light produced by the controlled lamp(s). The system can't directly measure the total illumination in the controlled area, but it can estimate the total illumination on the basis of the daylight level sensed by the photosensor and the known dimming level (or on/off state) of the lamps. It then adjusts the dimming level, or switches the lamps, to keep this estimated total illumination at the desired level.

Closed-loop systems are generally less expensive and easier to set-up than open-loop systems, and they inherently provide a lumen-maintenance capability to compensate for the aging of the lamps. However, they suffer from three disadvantages:
- Closed-loop systems typically require a greater number of photosensors per unit area: in general, a photosensor is required for each luminaire, which is not necessarily the case for open-loop systems.
- The only practical way to sense the light produced by a luminaire is with a downward-looking sensor—but the output of such a sensor depends on the reflectivity of the workplan, as well as on the luminous flux produced by the lamps. So changes in the workplane reflectivity (e.g. due to changes in the position or color of objects in the room) will affect the brightness of the lamps.
- Auto-switching (versus auto-dimming) is trickier to implement in a closed-loop system, and typically complicates the set-up process because the switching threshold depends on the on/off state of the lamps.
On the other hand, open-loop systems allow multiple luminaires to share a single daylight-sensing photosensor, and they work equally well in open-plan layouts and smaller rooms with a single luminaire. However, there are two primary disadvantages with open-loop operation:
- An open-loop system generally needs a relatively complicated commissioning process before it can accurately infer the daylight level under the controlled luminaire(s) from the photosensor signal—and it still may not operate as accurately as a closed-loop system under varying daylight conditions.
- Exploiting an open-loop system's ability to control multiple luminaires from a single sensor requires that the sensor and ballasts/relays be interconnected with a central controller, which significantly increase the cost and complexity of the installation. And even the simplest open-loop system with a single sensor and single ballast is more difficult to install than the typical closed-loop system, because its sensor must be mounted remotely (so that it doesn't "see" any of the light from the controlled luminaire).
An important consideration in the choice between closed-loop and open-loop control is the scale of the system: as discussed below, stand-alone single-zone or dual-zone systems are well-suited to closed-loop control, while integrated multi-zone systems can better exploit the advantages open-loop control.
Hybrid Closed-Loop/Open-Loop Control
Closed-loop and open-loop control can be used together advantageously in the same system.
One hybrid approach, described in the subsquent section, is to use closed-loop control for a "master" luminaire near a window, with a "slave" luminaire deeper in the room controlled open-loop from the same photosensor.
Another hybrid approach is to derive each luminaire's brightness control signal from both the interior and exterior illumination.
For example, as disclosed in U.S. patent 7,683,301 to Papamichael et al. (2010), the control signal can be derived by correlating changes in the outputs of inward-facing and outward facing photosensors. This gives the system the accuracy of closed-loop control, but ensures that it responds only to changes in daylight (as sensed by the outward-facing sensor)—thereby rendering it immune to changes in workplane reflectivity.
Integrated versus Stand-Alone Systems
Daylight-harvesting controls can be grouped into yet another pair of categories: integrated systems and stand-alone systems.
Integrated Systems
Integrated systems put one or more sensors and ballasts/relays under common control of an automation system that, often, also controls other building functions. Integration allows the sensors and control hardware/software to be shared among multiple ballasts/relays, offers the potential for additional savings through coordinated operation of the HVAC and lighting systems (and potentially even motorized window coverings), enables automated load-shedding during high-demand conditions, and allows the system's operation to be monitored and "tweaked" from a central location.
Because all the luminaires in an integrated system are already interconnected with a central controller, a single photosensor can be shared among multiple luminaires without need for additional wired or wireless links. Thus, integrated systems often take advantage of open-loop operation in order to reduce the number of required photosensors.
Stand-Alone Systems
Stand-alone systems are dedicated to control of one or two daylit zones and operate without connection to other building systems.
Single-Zone Systems
Single-zone systems control just a single group of nearby lamps. The most typical configuration is the fixture-dedicated system, which dedicates all of the functionality for single-zone control—including the photosensor—to a single lighting fixture/luminaire.
Because the photosensor is co-located with the lamps, fixture-dedicated systems provide closed-loop control, self-adjusting to maintain the desired lighting level. Closed-loop operation reduces the need for commissioning and inherently provides a lumen-maintenance function, while the co-location of all the components reduces labor costs. However, a typical window can illuminate more floor area than a single luminaire, so more than one single-zone system is typically needed to exploit all of the daylight:
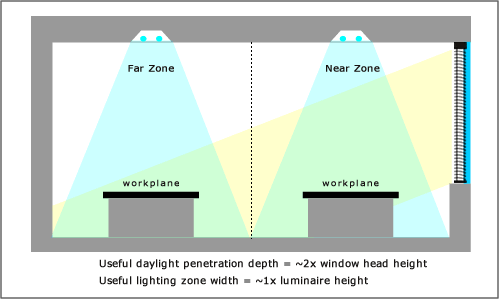
Dual-Zone Systems
A dual-zone system controls two adjacent luminaires, one in the "near zone" proximal to the window and one in the "far zone" deeper in the room. The objective is to use a single controller and photosensor to fully exploit all of the daylight from the window (or group of colocated windows), reducing cost relative to two single-zone systems.
Some dual-zone systems use open-loop control for both zones, but the most cost-effective systems (based on our own analysis) use a hybrid approach in which the near-zone luminaire has its own photosensor and operates under closed-loop control, while the far-zone luminaire operates under open-loop control. The open-loop control is typically performed under the assumption that the daylight level under the second luminaire is a fixed function of the daylight level under the first luminaire—a reasonable assumption unless direct sunlight penetrates the room.
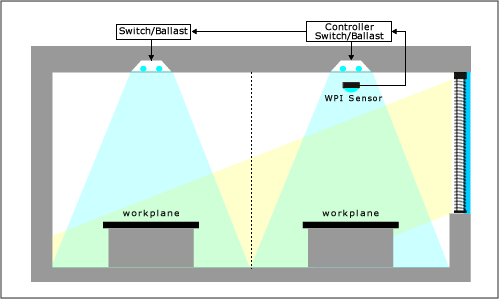
Cost
The decision to invest in energy efficiency is typically made on the basis of the simple payback period, defined as the cost attributable to the increased efficiency divided by the dollar value of the expected annual energy savings.
For daylight harvesting, the cost attributable to the increased efficiency depends on three factors beyond the installed cost of the equipment:
- whether the equipment offers benefits beyond daylight harvesting;
- why the equipment is being purchased (e.g. as an energy saving retrofit or for new construction), and
- whether the equipment qualifies for rebates or other incentives.
Benefits Beyond Daylight Harvesting
For systems that offer benefits beyond daylight-harvesting (e.g. manual dimming or automatic load-shedding capability), only a percentage of the total system cost is attributable to the daylight harvesting function. For example, if the system also provides a manual dimming control, then less than 100% of the cost of the dimming ballast should be allocated to the daylight-harvesting function, because manual dimming is a useful capability in its own right.
However, determining the percentage of multi-function system cost attributable just to daylight harvesting is inherently subjective. This is particularly true for integrated building-control systems, for which daylight harvesting is sometimes considered a secondary or "fall out" capability relative to the other functions.
For that reason, the costs discussed below are for stand-alone controls that provide only* daylight-harvesting functionality. This avoids the subjectivity associated with cost allocation, and provides a measure of conservatism because the cost of stand-alone controls is generally greater than the incremental cost of daylight harvesting in multi-function controls.
Reason for Purchase
The effective cost of an energy saving product depends on why it's being purchased:
- If it's being bought primarily to replace an installed device that's worn-out or obsolete—or for new construction or a major renovation—then the effective price is just the incremental installed price beyond a less efficient alternative product. This is the replacement/new construction scenario.
- On the other hand, if it's being bought primarily for energy savings—and if there's already a less-efficient installed device with signficant remaining life—then the effective price is the total installed price of the new product, plus the depreciated value of the existing product. This is the retrofit scenario.
The greater effective cost in the retrofit scenario weakens daylight harvesting's value proposition, making the retrofit market segment much harder to penetrate. However, because only a small fraction of non-residential floor area is newly constructed or renovated each year, the retrofit segment offers much greater near-term potential for revenues and energy savings. For that reason, only the retrofit scenario is addressed in the costs discussed below.
Incentive Programs
Incentive programs to stimulate energy efficiency have proliferated in recent years. Incentive programs are either prescriptive (in which a fixed benefit is provided for each prescribed energy saving technology or measure), or savings-based (in which the benefit is based on the actual kW or kWh reduction).
Most prescriptive programs explicitly list daylight-harvesting equipment among the qualifying technologies, and some also offer a "custom" provision that can provide benefits for any demonstrably effective energy saving technology. And some prescriptive programs provide benefits based on LEED points, which can be earned by daylight-harvesting lighting controls.
The result is that most daylight harvesting projects will qualify for some sort of rebate, tax deduction, or tax credit, significantly reducing the effective cost.
However, because there is no guarantee that such incentives will be offered indefinitely, costs are reported herein for the no-incentive scenario as well as for typically available incentives. The assumed incentives are limited to utility rebates, which are widely available and presumably more likely to persist in the long-term than tax incentives.
Installed Cost of Equipment
The installed cost of daylight harvesting equipment varies over a wide range depending on the type and complexity of the installation. However, as previously mentioned, the costs quoted herein are exclusively for stand-alone controls that provide only daylight harvesting functionality, and the variance in installed cost of such controls is relatively small.
For the same reason, the costs quoted below are further limited to fixture-integrated stand-alone controls in both single-zone and dual-zone configurations. Fixture-integrated controls are arguably the purest form of daylight-harvesting control because they have the smallest hardware footprint and provide no additional capability beyond daylight harvesting (other than lumen maintenance).
Fixture-integrated controls consist of a controller/switch, attached photosensor, and one or both of a dimmable ballast or switching relay. Some fixture-integrated controllers also require a separate low-voltage power supply, but the costs quoted herein are for controllers with integral power supply.
The prices herein are for specific makes/models of lighting control, and were based on single-quantity prices quoted on the web. A discount of 33% was then subtracted to estimate the per-unit price for large retrofit projects.
Commissioning Labor
One of the market issues with daylight-harvesting lighting controls is that they frequently fail to provide the expected savings, which is often attributed to inadequate commissioning (i.e. tuning and testing by specially qualified personnel before being placed into service).
However, our own analysis indicates that inadequate commissioning is a smaller issue for sidelit applications than three other causes of poor performance: inadequate control algorithms, improper specification/installation (e.g. poor selection of photosensor mounting location or orientation), and—especially—over-shaded windows.
Further, the latest daylight harvesting lighting controls need much less commissioning than previous-generation controls, and some products require virtually no commissioning in order to achieve full performance. This is the case for the simple lighting controls assumed herein.
However, for the sake of conservatism, the installed costs given herein do include commissioning labor.
Cost per Square Foot
The most convenient cost metric for purposes of estimating daylight harvesting project costs and payback periods is the cost per square foot, rather than the unit cost (i.e. the cost per lighting control). For fixture-integrated lighting controls, the cost per square foot is equal to the unit cost divided by the illuminated area per luminaire.
Based on a web search and an informal survey of buildings in the Washington, DC metro area, the most frequently used luminaire configuration in existing office buildings is a two-foot by four-foot (2x4) recessed parabolic troffer with three standard T-8 lamps and a first-generation electronic ballast (for a total luminaire power requirement of 88 W), mounted on an 8-foot by 10-foot grid. This is also the same configuration generally assumed as the base-case in recent studies investigating lighting system upgrades. This 88-W 80-sf configuration has an installed Lighting Power Density (LPD) of 1.1 W/sf, which is equal to the mode of the LPD distribution assumed in our savings estimates.
Other linear-fluorescent luminaire configurations include two and four-lamp 2x4's, two-lamp 2x2's, and single-lamp recessed and suspended fixtures; other luminaire spacings include 8x8, 10x10, and 10x12 feet. However, the 8x10 spacing appears dominant in existing office buildings, and appears close to the median and mean of what seems to be a roughly normal distribution of areas-per-luminaire in existing buildings.
Therefore, our cost estimate is based on median illuminated area of 80 square feet per luminaire, yielding an assumed floor area of 80 square feet for a single-zone system and 160 square feet for a two-zone system.
The following chart summarizes the retrofit cost per square foot for the daylight-harvesting lighting controls considered here, with and without typical rebates:
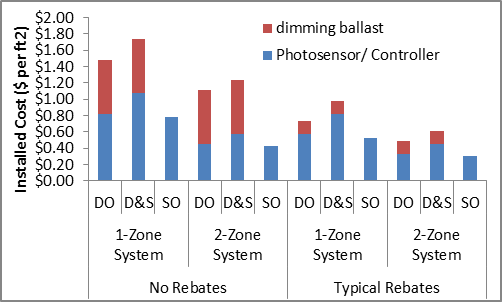
Savings
The energy savings (kWh) provided by daylight harvesting is equal to the integral of the instantaneous power savings (W) over time.
Relative Power Savings
The relative (percentage) reduction in lighting power depends on the daylight level relative to the set-point. The following chart shows how the relative power savings vary with instantaneous daylight level for each type of lighting control:
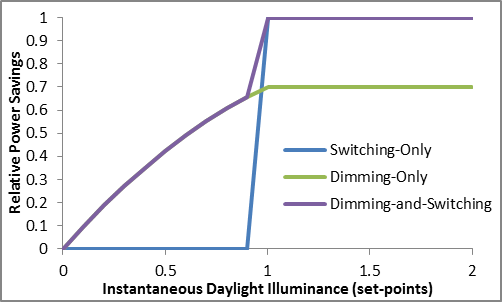
Switching-only controls provide no power savings when the daylight level is below the set-point, but provide 100% savings when the daylight level is above the set-point. On the other hand, while dimming-only controls are able to provide some savings at lower daylight levels, the maximum savings at higher daylight levels are limited to about 70% due to dimming inefficiencies. The dimming-and-switching controls provide the greatest power savings over the whole range of daylight levels.
Power savings are important not just because they influence energy savings, but also because they can reduce peak electrical demand, which in turn reduces utility demand charges. For some buildings, the savings due to reduced peak demand charges can be a substantial fraction of the overall dollar savings from daylight harvesting.
Relative Energy Savings
The relative energy savings (e.g. the integral of the power savings over time) in a particular installation depend on power savings curves of Chart 6, as well as on how the horizontal daylight illuminance on the workplane varies over time relative to the lighting control set-point.
If the daylight illuminance exceeds the set-point for most of the day (e.g. in areas close to windows on sunny exposures), then the average annual energy savings can exceed 75% for dimming-and-switching controls. On the other hand, if the daylight illuminance rarely exceeds the set-point (e.g. in areas further away from windows on northern exposures), then the average energy savings can be less than 25%. The following chart shows how the average relative savings vary with the time-averaged daylight illuminance (relative to the lighting control set-point) in a typical sidelighting installation in the continental USA:
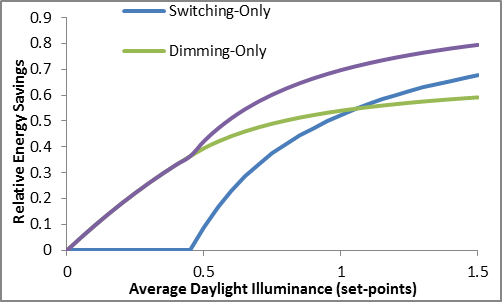
In any given installation, the average daylight illuminance—and, hence, the average power and energy savings—depend on distance from the window as well as the degree to which the window is shaded. Referring to Chart 8, the average daylight illuminance with unshaded windows with sunny (non-north-facing) exposures in the continental USA is about 1.0 lighting set-points (i.e. about 500 lux) over the entire sidelit area (ranging up to 2.0 window heights from the window) and 1.6 set-points (i.e. about 800 lux) in the near zone (ranging up to 1.0 window heights from the window).
However, within the effective sidelit area (up to about 2 window heights from the window), the average daylight level depends much more on the degree of window shading than on distance from the window. Shading can drastically reduce the average daylight level, and hence the average savings. For example, the savings 2 window heights from an unshaded window can be much greater than the savings 1 window height from a shaded window.
As shown in Chart 8, switching-only controls can provide greater savings than dimming-only controls with unshaded windows in the near zone, but dimming-only controls provide greater savings than switching-only controls over the entire sidelit area—especially if the windows are shaded. In fact, if shading drops the average daylight level below about 0.5 set-points, then switching-only controls provide no savings at all.
Absolute Savings
Daylight harvesting's absolute power and energy savings (in W and kWh, respectively) depend on the installed Lighting Power Density (LPD) and annual lighting Energy Use Intensity (EUI), respectively, as well as on the relative savings discussed above. In conjunction with the local utility rate structure, these absolute power and energy savings then determine the absolute dollar savings.
The various installation-specific variables that affect these absolute savings—i.e. the degree of window shading (which heavily influences the average daylight level, and hence the relative savings), the LPD, and the average number of lighting hours per year (which, along with LPD, determines the annual lighting EUI), and local utility rates—result in a broad distribution of expected dollar savings across the market. That distribution is heavily influenced by the fact that sidelit areas in existing buildings are typically over-shaded, significantly reducing the savings from daylight harvesting
For example, the following chart shows the projected median dollar savings for selected daylight-harvesting lighting controls in retrofit applications, taking into account the actual distribution of window shading settings:
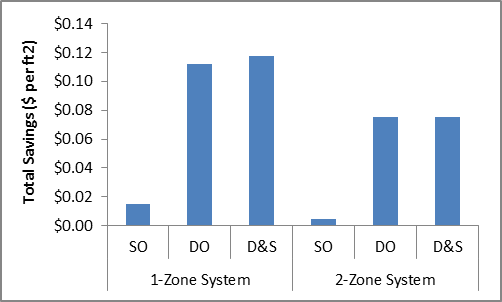
As shown in Chart 9, the presence of window shading in typical sidelit areas reduces the average daylight level enough to slash the savings of switching-only controls to near zero—especially in a dual-zone configuration. The other controls are affected too, but to a lesser degree.
Payback Period
The costs of stand-alone daylight-harvesting lighting controls vary over a narrower range than the energy savings, so the payback periods are very nearly proportional to the reciprocals of the dollar savings bars of Chart 9. The following chart shows the projected median paybacks across the entire market, again taking into account the distribution of window shading settings:
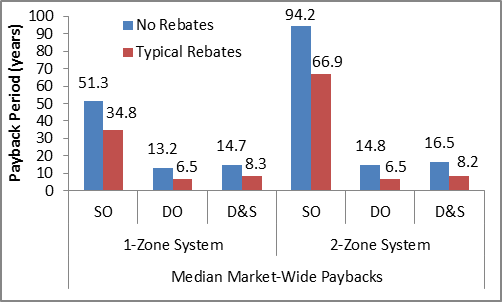
As evident from Chart 10, the median paybacks aren't competitive with those of mainstream retrofit technologies, due primarily to the prevalence of over-shaded windows.
Of course, the paybacks will be much shorter than these median paybacks in the small percentage of sidelit areas receiving plenty of daylight. In fact, the savings are so sensitive to the average daylight level that any one of the six configurations of Chart 10 (with the exception of switching-only controls in a two-zone configuration) could achieve the shortest payback in a given installation.
Summary of Key Points
- Today's daylight-harvesting lighting controls span a broad range of cost and capability
- One important design variable affecting cost and performance is the granularity with which the lighting power is controlled:
- Switching-only controls are the least expensive, but require high average daylight levels to save significant amounts of energy
- Dimming-only controls provide the smoothest, least-perceptible lighting adjustment, but cost more than switching-only controls and save less energy when the average daylight level is high
- Dimming-and-switching controls provide the greatest energy savings, but also have the highest cost
- Other important variables include the control protocol (open-loop versus closed-loop) and integrated versus stand-alone configurations. One of the most cost-effective configurations is a stand-alone two-zone system with closed-loop control of the zone nearest to the window, and open-loop control of the deeper zone
- Installed cost for stand-alone controls ranges from $0.30 to $1.70 per square foot, depending on the configuration and availability of utility rebates
- Savings are highly sensitive to the average daylight level in the sidelit space. Due to window over-shading, median market-wide savings for switching-only controls are neglible, while savings for the other types of control range are of the order of just $0.10 per square foot
- For the same reason, median market-wide retrofit paybacks for switching-only controls exceed 20 years, while those of the other controls range from 4 to 15 years
- Savings are much greater, and paybacks much shorter, in the small percentage of sidelit areas receiving plenty of daylight